The Project
Driving Innovation in Light-Matter Interaction and Hybrid Systems
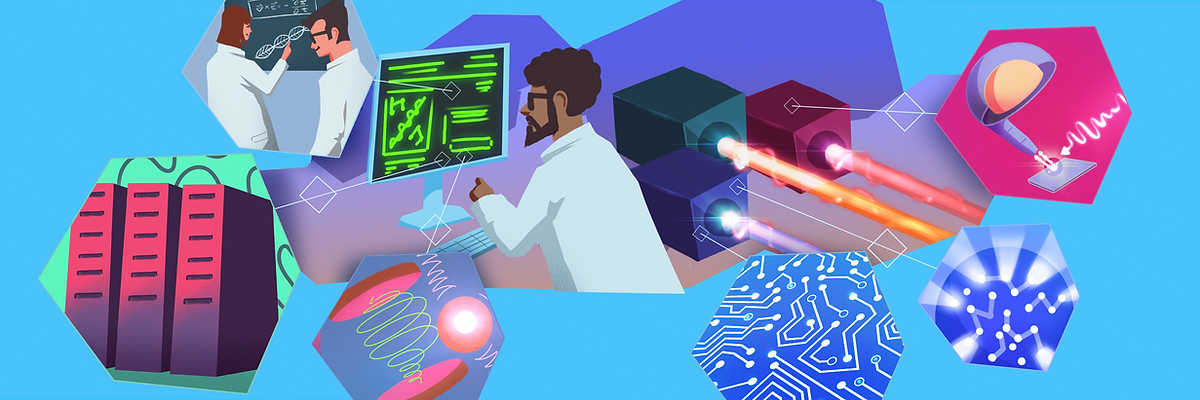
Introduction
SPARKLE is at the forefront of research in polaritonics and light-matter hybrid systems. By combining state-of-the-art experimental methods with advanced theoretical simulations, the project provides Europe with its first generation of 14 highly trained early-stage career scientists.
These researchers will gain multidisciplinary training across theoretical solid-state physics, quantum optics, ab initio material simulations, photonic engineering, laser physics, and ultrafast spectroscopy. SPARKLE follows the guiding principle of “model - simulate - design - measure”, ensuring a comprehensive and integrated approach to light-matter research.
By equipping scientists with entrepreneurial, organizational, and management skills, SPARKLE prepares future leaders ready to excel in academic and industrial environments.
Research Topics
EXPERIMENT
Innovative Light Sources
CNR | EPFL
Our research spans both theoretical and experimental approaches to light-matter interactions, with key topics including theoretical modeling of quantum materials, experimental exploration of light-dressed properties, development of advanced spectroscopies, and the creation of tailored light sources.
THEORY
Out-of-Equilibrium Theories
UniPa | JSI
EXPERIMENT
Experimental Spectroscopies
CNR | CNRS
THEORY
Ab-Inito Materials Simulations
DTU | FAU | BIU
EXPERIMENT
Optical System Design
EPFL | FAU | BIU
THEORY
Electrodynamics Simulations
UniStra | CSIC
Objectives
SPARKLE’s research objectives focus on harnessing light to design and control material functionalities in ways that are independent of chemical modifications. These are our research objectives (RO).
RO1
Symmetry-Driven Quantum Material Engineering
Exploit light to engineer phases in quantum materials, by (i) tailored laser waveforms for programmable elec- tronic topology; and (ii) spatially engineered photonic environments to break electronic symmetries. To pinpoint the new quantum phases through novel theory-informed spectroscopies and transport measurements, e.g., anomalous quantum Hall effect.
RO2
Emergent Properties in Light-Dressed Materials
Leverage realistic Floquet-based theoretical simulations to enhance the light-matter coupling and im- implement novel polaritonic spectroscopies to assess the light-dressing of material excitations. This will enable us to thoroughly treat the electron-phonon-photon coupled problem and guide proof-of-concept experiments demonstrating the light-induced metal-insulator transitions via cavity-mediated vibrational interactions.
RO3
Advanced Spectroscopies and Tomography
Adapt the design of spectroscopic tools such as Angle-Resolved Photoemission Spectroscopy (ARPES), X-Ray, and Magnetic spectroscopies to detect polaritons on ultrafast time scales while allowing to assess their quantum nature. This will then be paired with developing ab-initio models to rationalize the observation of novel polaritonic phenomena. Implement ab-initio ARPES to visualize light-dressed electronic orbitals28 and develop theoretical spectroscopies for polaritons of a magnetic nature.
RO4
Generation of tailored light
Develop multicolor laser pulses, tunable THz sources, integrated photonic circuits for sensing cavity quantum fields, efficient random lasers with halide perovskite materials, and explore quantum phases as sources for correlated photons.
Objectives
SPARKLE’s research objectives focus on harnessing light to design and control material functionalities in ways that are independent of chemical modifications. These are our research objectives (RO).
RO3
Advanced Spectroscopies and Tomography
Adapt the design of spectroscopic tools such as Angle-Resolved Photoemission Spectroscopy (ARPES), X-Ray, and Magnetic spectroscopies to detect polaritons on ultrafast time scales while allowing to assess their quantum nature. This will then be paired with developing ab-initio models to rationalize the observation of novel polaritonic phenomena. Implement ab-initio ARPES to visualize light-dressed electronic orbitals28 and develop theoretical spectroscopies for polaritons of a magnetic nature.
RO1
Symmetry-Driven Quantum Material Engineering
Exploit light to engineer phases in quantum materials, by (i) tailored laser waveforms for programmable elec- tronic topology; and (ii) spatially engineered photonic environments to break electronic symmetries. To pinpoint the new quantum phases through novel theory-informed spectroscopies and transport measurements, e.g., anomalous quantum Hall effect.
RO2
Emergent Properties in Light-Dressed Materials
Leverage realistic Floquet-based theoretical simulations to enhance the light-matter coupling and im- implement novel polaritonic spectroscopies to assess the light-dressing of material excitations. This will enable us to thoroughly treat the electron-phonon-photon coupled problem and guide proof-of-concept experiments demonstrating the light-induced metal-insulator transitions via cavity-mediated vibrational interactions.
RO4
Generation of tailored light
Develop multicolor laser pulses, tunable THz sources, integrated photonic circuits for sensing cavity quantum fields, efficient random lasers with halide perovskite materials, and explore quantum phases as sources for correlated photons.
Research Topics
Our research spans both theoretical and experimental approaches to light-matter interactions, with key topics including theoretical modeling of quantum materials, experimental exploration of light-dressed properties, development of advanced spectroscopies, and the creation of tailored light sources.
Experiment
Innovative Light Sources
CNR | EPFL
Experiment
Experimental Spectroscopies
CNR | CNRS
Experiment
Optical System Design
EPFL | FAU | BIU
Theory
Electrodynamics Simulations
UniStra | CSIC
Theory
Out-of-Equilibrium Theories
UniPa | JSI
Theory
Ab-Inito Materials Simulations
DTU | FAU | BIU

Use classical light to explore polariton formation, non-equilibrium phases, and their applications in light-controlled magnetism and Floquet-topology. Within WP1, our focus is on precise excitation and manipulation of nuclear, electronic, and magnetic structures, striving for ultrafast control over material functionalities.
In this WP we harness and study quantum light's fluctuations confined within structured photonic environments to influence material equilibrium phases.
We develop experimental and theoretical tools for designing photonic environments, assessing field fluctuations, and creating correlated light using tailored materials. WP2 complements WP1, expanding our understanding of light-matter interactions into the quantum realm.
This WP advances spectroscopic techniques to examine light and electron interactions in complex light-matter hybrid systems. WP3 focuses on developing advanced spectroscopic methods, such as ultrafast pump-probe optical spectroscopy and time-resolved ARPES, and gaining a microscopic view into our engineered materials' inner workings.
Here, we ensure that tasks are executed and milestones are reached according to plan. The project results will be communicated and disseminated by the DCs to the general public, commercial stakeholders, and academic community through means and channels defined in WP5
All DCs will receive extensive scientific courses and transferable skills training (e.g., entrepreneurship, project management).
This WP ensures top-of-the-class preparation for both academic and non-academic careers.
This WP implements principles of equality among gender and cultural diversity, setting up an inclusive culture across the whole DN.